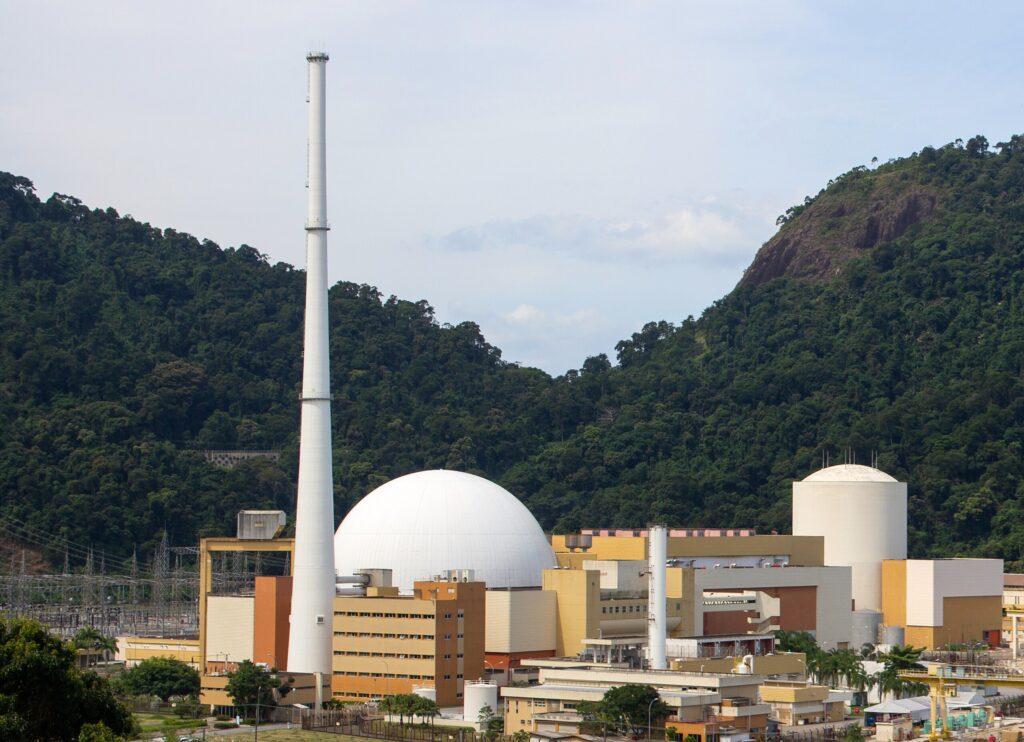
At some point, Australian voters are going to be asked to vote upon (amongst other things) whether or not we would like to remove the ban on nuclear power in Australia. This blog is intended to give some technical detail related to nuclear power and some of the issues that voters might like to take into consideration.
Although I have my own political and commercial biases (and in the interests of full disclosure, I support removing the ban and Arche Energy would seek to become involved in an Australian nuclear project), I’m going to try to be as factual as possible, try to stay clear of politics and I won’t compare nuclear to other forms of energy.
Feel free to comment (and correct where necessary), but please keep it technical and polite (I’m not a politician).
Why bother?
The primary value proposition for nuclear energy (in the context of energy transition) is to provide a large volume of base load (i.e. capable of operating on a 24/7 basis), lower cost energy that has a very low carbon intensity.
The second value proposition is that, if similar sized nuclear power plant are located in the same place as retiring base load coal plant, then the existing transmission, water and civil infrastructure can be reused; thereby avoiding new build infrastructure and the related capital, resumption of land, land clearing and impacts on communities.
Nuclear’s other comparative advantages include a very low land use intensity (Ha/MW) and very low volumes of waste.
How does a nuclear power plant work?
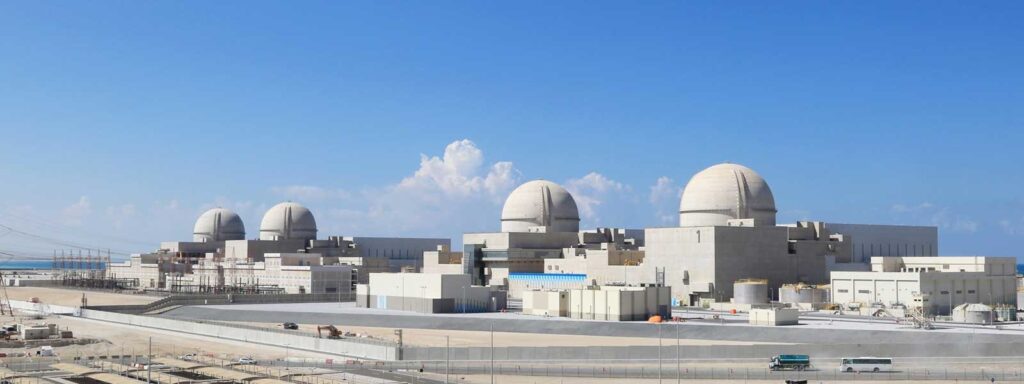
Although there are many forms of nuclear power (a topic for another blog), for the purposes of this blog I am going to talk about nuclear fission and focus on pressurised water reactors such as the APR1400 recently commissioned at Barakah Nuclear Power Plant in the Emirate of Abu Dhabi (on the rationale that, although the unit size is a bit big for Australia, it is a recent build from a contractor (KEPCO E&C) who I would seriously consider for construction in Australia).
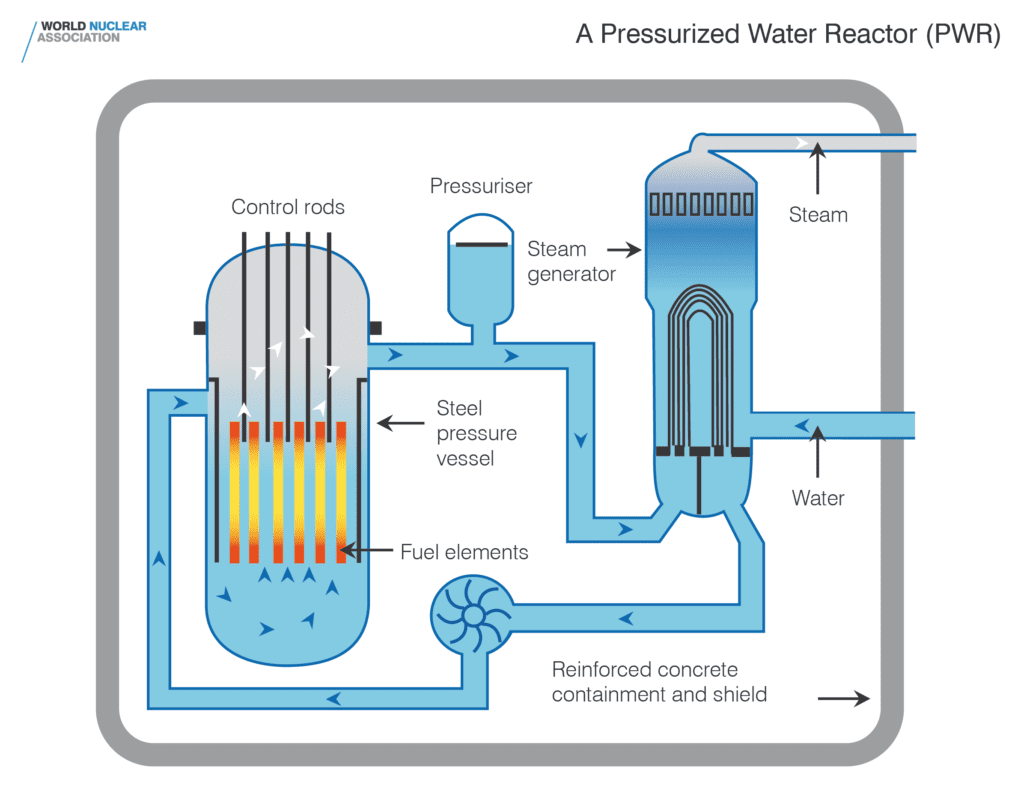
A pressurised water reactor is a light water reactor, that is it uses regular H2O to transfer heat from the nuclear core (rather than heavy water, deuterium oxide, which is still H2O, but the hydrogen (deuterium) has a neutron in its nucleus (and an atomic weight of two), whereas bog standard hydrogen does not).
The water is pressurised to allow heat transfer at higher temperatures (323.9oC) without the water flashing off into steam (allowing higher heat transfer rates).
The energy starts in the fuel rods, containing uranium dioxide. The uranium is a low enriched blend of 3-5% U235 (an isotope of uranium with an atomic weight of 235 – 92 protons and 143 neutrons), with the balance being U238 (which is not fissionable).
The fuel rods are separated by control rods, these control rods are capable of absorbing neutrons and control the rate of the nuclear reaction.
To start the reaction control rods are removed and neutrons (from a neutron source) are shot into the fuel rods. When a neutron hits a U235 atom, it becomes U236, which is unstable and splits into two other atoms (Barium and Krypton) producing heat (and lots of it) and also another two neutrons (now there are three neutrons kicking around the reactor). These three neutrons then bump into another three U235 atoms and thus the chain reaction propagates.
The control rods absorb neutrons (that is they stop them from bumping into new U235 atoms) and are moved up and down the column of fuel rods to control the reaction speed. When the control rods are fully inserted, the reaction becomes sub-critical and eventually stops (although decay heat must still be removed from the core for some time after the reaction ceases).
Heat from the reactor core is removed using the pressurised water, this water circulates in a closed loop to a steam generator, where the heat is used to generate steam. The steam then powers a turbine similar to that used in a coal fired power plant (although optimised for the lower temperatures and pressures used in nuclear processes).
Small Modular Reactors (SMR)
As I understand it, to date, only Russia, India and China have operational SMRs.
Small modular reactors (SMR) are/will be small (e.g. 300 MW or lower) and are/will be manufactured in a modular arrangement. They use the same core technology as large reactors, just smaller. The modular manufacturing process is intended to reduce time and cost of production.
The GE Hitachi BWRX-300 will be a 300 MW single unit reactor, the first of which is being planned for deployment to Darlington New Nuclear Project in Ontario, Canada in 2028.
Rolls Royce have executed a number of MoUs for the deployment of small modular reactors in Finland, Sweden and Ukraine.
Canada’s CANDU reactors are modular, but not small and are a very mature technology) with the first 600 MW units being deployed at Pickering in 1971.
The Chinese ACP100 is a 126 MW SMR which is currently in the construction phase.
300-700 MW is a good unit size for the NEM (similar to existing coal fired units) and by the time an Australian project is at the technology selection stage (mid 2026: see timeframe assumptions below), some of these early SMRs will have some real world construction, commissioning and operating experience.
Cost
I have three sources for capital cost of Barakah: $20Bn, $24Bn and $32Bn USD for the four x 1,345 MW units. I’m going to use $24Bn USD for the purposes of this blog. With a WACC of 10%, exchange rate of 0.7, a life of 60 years and availability of 90%, this equates to a capital cost of $81.10/MWh (AUD).
If I use a uranium price of $40US per pound, this equates to around $6USD per MWh as a fuel cost: $8.57/MWh AUD.
If you then allow 5% of capex as the annual non-fuel opex and cost of dry cask storage, you get $40.42/MWh AUD. This is a pretty crude assumption, so if you have better data, please drop me a note.
This sums to $130.9/MWh. You can probably add a small premium to account for Australia’s higher construction costs and the smaller sized units suitable for Australia.
If I use a WACC of 5% and non-fuel opex of 3%, I get $75.52/MWh.
If I use the higher estimate of $32Bn US, I get $171/MWh.
The $130/MWh estimate is comparable with the annual average spot price in most NEM zones for the financial years ending June 30 2022 and 2023: ($84 to $162/MWh).
While this estimate is higher than reported LCOEs for solar and wind generation, to make a fair comparison you also have to allow for the cost of firming and/or storage and pioneering infrastructure (e.g. renewable energy zones and related transmission).
The Minerals Council of Australia, “SMRs, Small modular reactors in the Australian context“, Second Edition estimates $64-77/MWh and CSIRO’s GenCost report estimates $282/MWh in 2030; so it is fair to say that there is a range of disagreement (and uncertainty) on cost.
In my view, until we do a real feasibility study for a real project with real developers and investors and the strong and enthusiastic support of whomever is conducting the study, we won’t see a reliable and robust competitive cost estimate for nuclear power in Australia.
Time
Construction of Barakah Unit 4 (the most recently completed unit) commenced on 30 July 2015 and was completed in December 2023; a construction timeframe of 8.5 years. Units 1, 2 and 3 each all had a similar construction timeframe.
French pressurised water reactor (950 MW) construction timeframes range from 50 to 90 months (4.2 to 7.5 years) and the the world wide construction timeframe correlates with size (that is smaller ones are faster). Ontario Power Generation are aiming for a five to six year build on a 300 MW small modular reactor at Darlington.
In the context of an Australian project, a smaller, 300 MW scale plant is about right and assuming nth of a kind (i.e. a mature technology and experienced construction workforce) and modular construction, a five year build time is probably a reasonable estimate.
In the context of a new nuclear plant replacing an existing coal plant without clearing or new connecting infrastructure and dry cask storage of waste on site prior to reprocessing and with an assumption of willing and enthusiastic government support, I would allow a timeframe of around three years to complete an EIS and FEED.
I have excluded any schedule delays due to appeals, court actions and government related delays (the reader can add on whatever contingency they want to allow for these potential delays).
So, if the ban was lifted shortly after the next federal election (say late 2025) and the relevant state is willing and able to assess an EIS for a nuclear plant by late 2026, then we might see financial close on a project from late 2028 and electrons flowing from late 2033 (about ten years from today).
In the context of a major infrastructure project such as a base load power station, a ten year development timeframe is reasonable.
Fuel and enrichment
Australia holds almost one third of the world’s uranium and, in 2022 was the world’s forth ranked producer. Clearly fuel supply from our internal resources will not be a problem; however, Australia does not have enrichment facilities.
Light water reactors require enrichment of uranium to around 3-5% U235. Natural uranium contains around 0.72% U235.
I won’t go into the various methods of enrichment (James Krellenstein gives a great description to Dr Chris Keefer in this Decouple podcast) aside to say that it is capital and energy intensive and most of the current global enrichment capacity is in Russia.
The Canadians use a pressurised heavy water reactor, the CANDU reactor. The heavy water reactors do not require enriched uranium, which makes Canada independent of foreign enrichment services. This strategy might be a good idea for Australia with respect to energy independence.
Australian company Silex Systems are developing an enrichment process using lasers here in Australia. Irrespective of the ban on nuclear power generation; an enriched uranium sourced from Australia and enriched in Australia with Australian technology would be well regarded by our geopolitical friends and would be a great replacement export for fossil fuels.
Waste
Nuclear plant produces waste. 3% of this waste is high level waste (spent fuel rods). 7% is intermediate level waste (e.g. filters, steel components and some effluents). 90% is low level waste (e.g. tools, gloves, PPE). On average, the waste from a reactor supplying a person’s electricity needs for a year would be about the size of a brick. Only 5 grams of this is high-level waste – about the same weight as a sheet of paper.
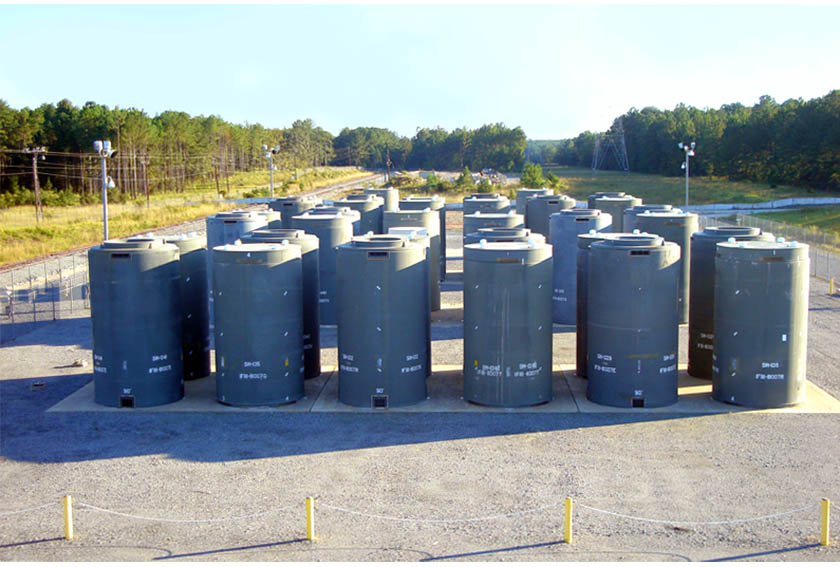
High level waste is cooled in wet storage (basically a large swimming pool) and then transferred to dry casks. These casks can then be stored pretty much anywhere and are typically just warehoused or stored on laydown areas.
The high level waste are solid fuel rods (not leaking drums of glowing green liquid) and the casks are incredibly strong (designed to survive truck and rail accidents) and are very effective radiation shields.
Spent fuel rods contain approximately 95% of their original energy and can be reprocessed into new fuel rods.
Intermediate and low level waste is generally disposed of in controlled landfill, with some intermediate level waste requiring shielding.
Safety
Conservative engineering and higher standards
My power station mates will quickly point out that the steam temperatures are actually a pretty low compared to what we can do with an ultra-super critical coal plant (and the thermal efficiency will suffer as a result). The nuclear industry does not push the boundaries of metallurgy the way that we do in coal plant so that they can use more robust materials which are less susceptible to failure such as creep and stress corrosion cracking.
In the way that aircraft engines are more carefully looked after than tractor engines, the nuclear industry also has more robust, thorough and conservative maintenance and asset management practices than the conventional power generation industry.
Modern safety systems
Modern small modular reactors utilise passive safety features such as natural circulation, convection, gravity and self-pressurisation.
A convective cooling system involves arranging the reactor core and heat exchangers as a thermosiphon so that hot water or steam from the core moves upwards to heat exchanger and the cooler water from the heat exchanger (being more dense) then drops to the bottom of the reactor vessel.
Control rods in modern plant are held up by electro-magnets rather than mechanical linkages, so that if there is a power failure the control rods will be released and drop to the bottom of the reactor.
Modern nuclear plant are designed to withstand complete power outages, for example the APR1400 has a blackout coping time of eight hours and GE Hitachi claim that the BWRX-300 can cool itself (using passive cooling) for seven days without power or operator action.
The CANDU system has a fail safe system that drops the control rods into the reactor core very quickly (under gravity) to stop the reaction and a liquid neutron absorbing poison that immediately stops the reaction.
Fukushima Daiichi
We can’t have a conversation about nuclear energy without considering Fukushima and Chernobyl.
Fukushima Daiichi consisted of six GE light water boiling water reactors of 1960s design (a boiling water reactor is similar to a pressurised water reactor except that steam is generated in the reactor vessel rather than in a separate steam generator). The 15m tsunami hit the power station and power was lost to all duty, standby and emergency pumping systems resulting in a loss of circulating water to cool the reactor vessel and (the short version) eventually lead to core meltdown on three of the units.
In the context of an Australian nuclear power plant constructed with modern passive safety systems: while it is almost impossible that a tsunami would hit an Australian power station, loss of electrical power for other reasons (including flooding) is possible. However, with a robust HAZOP (identifying the risk of flood impacting the emergency generators) and modern passive cooling systems, it is unlikely that a Fukushima Daiichi event would occur.
Chernobyl
Chernobyl Unit 4 was an RBMK reactor. The operators were conducting a test of the steam turbine’s ability to power (using the steam turbine’s inertia alone) the emergency feedwater pumps in the event of a simultaneous loss of external power and coolant pipe rupture. During the test, the plant was in an abnormal, manually controlled operating condition (importantly, the control rods had been extracted manually. As the turbine’s speed dropped the water flow rate decreased (less power from the turbine to the feedwater pumps) and steam voids started to form in the reactor.
Meanwhile the reactor was operating without moderation from the control rods. The operators hit the AZ-5 button (emergency shut down) and all control rods began to be inserted at a rate of 0.4 m/s. The RBMK control rod has a graphite neutron moderator section attached to its end to boost reactor output by displacing water when the control rod section had been fully withdrawn from the reactor, so the impact of inserting the control rod initially increased the reaction rate. A power spike then occurred, the control rods fractured and the rest is history.
In the context of an Australian nuclear project with a passive cooling system and multiple redundant power supplies to feedwater pumps, it would not be necessary to undertake these types of tests (that’s not to say that the test was necessary at Chernobyl, it seemed like a bit of an unnecessary experiment to me). With an electromagnet suspended control rod system, the control rods would have reacted faster and the reaction slowed more quickly.
Skills
We have some excellent engineers, technicians and tradespeople here in Australia, so let’s not sell ourselves short. We are good at managing complex and dangerous production facilities.
Yes we will need skilled and experienced workers from overseas (but we need these for pretty much every major project that we do and lots of clever engineers want to move to Australia).
We won’t be the first movers and so we will be able to draw in skilled people from other projects.
With the exception of the reactor and fuel handling plant, the remaining plant at a nuclear power station is very similar to a coal power station and we most certainly have some excellent thermal plant operators, maintainers and engineers in the industry.
Most of us engineers studied physics at university and with the benefit of OEM training and support, would be more than capable of operating and maintaining a small modular reactor based power plant.
We also have the benefit of the skills and knowledge of ANSTO operating the OPAL reactor at Lucas Heights.
Final thoughts
The topic is complex and there are a mix of political and commercial biases that will influence debate. My hope, is that most voters will spend the time to understand the topics before making an informed voting decision. I hope I haven’t let my personal biases come through too much.
Our votes are valuable, let’s not waste them with ignorance, group think or lazy thinking.
If you have any constructive comments, corrections or clarification, please reach out.
About the author
Andrew is an engineer and the founder of Arche Energy with 28 years’ experience in the energy industry, primarily in power generation.